The study also sheds light on the long-standing neuroscience mystery known as the “credit assignment problem”—how individual synapses, which only have access to local information, manage to support the brain’s broader learning processes
In a groundbreaking study, neurobiologists have revealed how learning changes the brain’s circuitry, offering valuable insights that could reshape our understanding of neurological disorders and artificial intelligence systems.
The research, published on April 17 in the journal Science, was led by William “Jake” Wright, Nathan Hedrick, and Takaki Komiyama of the University of California, San Diego. Using cutting-edge visualisation technologies such as two-photon imaging, the team was able to observe brain activity at an unprecedented level, tracking the behavior of synapses and neurons in live mice as they learned new tasks.
At the core of this study is the concept of synaptic plasticity—the process by which synapses, the connections between neurons, strengthen or weaken in response to new information. This process is fundamental to how we learn and retain everything from song lyrics to complex work routines. Until now, scientists believed synaptic plasticity followed a single set of rules throughout the brain. However, the UC San Diego team discovered that this assumption oversimplifies the complex nature of how neurons adapt. Their observations showed that individual neurons follow multiple, distinct rules, depending on their specific location and function. This finding challenges conventional wisdom and provides a clearer picture of how learning and memory actually work.
“When people talk about synaptic plasticity, it’s typically regarded as uniform within the brain,” explained Wright, the study’s lead author and a postdoctoral scholar at the School of Biological Sciences. “Our research provides a clearer understanding of how synapses are being modified during learning, with potentially important health implications, since many diseases in the brain involve some form of synaptic dysfunction.”
The implications of these findings are vast. On the neurological front, the discoveries could provide fresh approaches to treating conditions like addiction, post-traumatic stress disorder, Alzheimer’s disease, and autism. Since these disorders often involve disruptions in synaptic plasticity, understanding how different synapses follow different plasticity rules could lead to targeted treatments that are more effective and personalised.
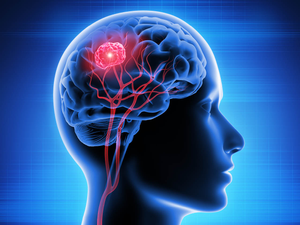
The study also sheds light on the long-standing neuroscience mystery known as the “credit assignment problem”—how individual synapses, which only have access to local information, manage to support the brain’s broader learning processes. The researchers likened this to individual ants working on specific tasks without knowing the colony’s bigger goals. Understanding how this decentralised system coordinates to produce learned behaviour could help explain some of the brain’s most complex functions.
Beyond health, the findings are generating excitement in the field of artificial intelligence. Traditional AI neural networks rely on a uniform set of learning rules across all units. However, this research suggests that diverse plasticity rules within a network may improve performance and flexibility, potentially leading to the development of more advanced and brain-like AI systems.
The study was supported by several National Institutes of Health (NIH) research and training grants, underscoring its scientific importance and broad potential. As scientists continue to explore the intricacies of brain function, this study marks a significant leap forward in understanding how we learn—and how this understanding can be harnessed to improve both technology and human health.